Hydrogen Production
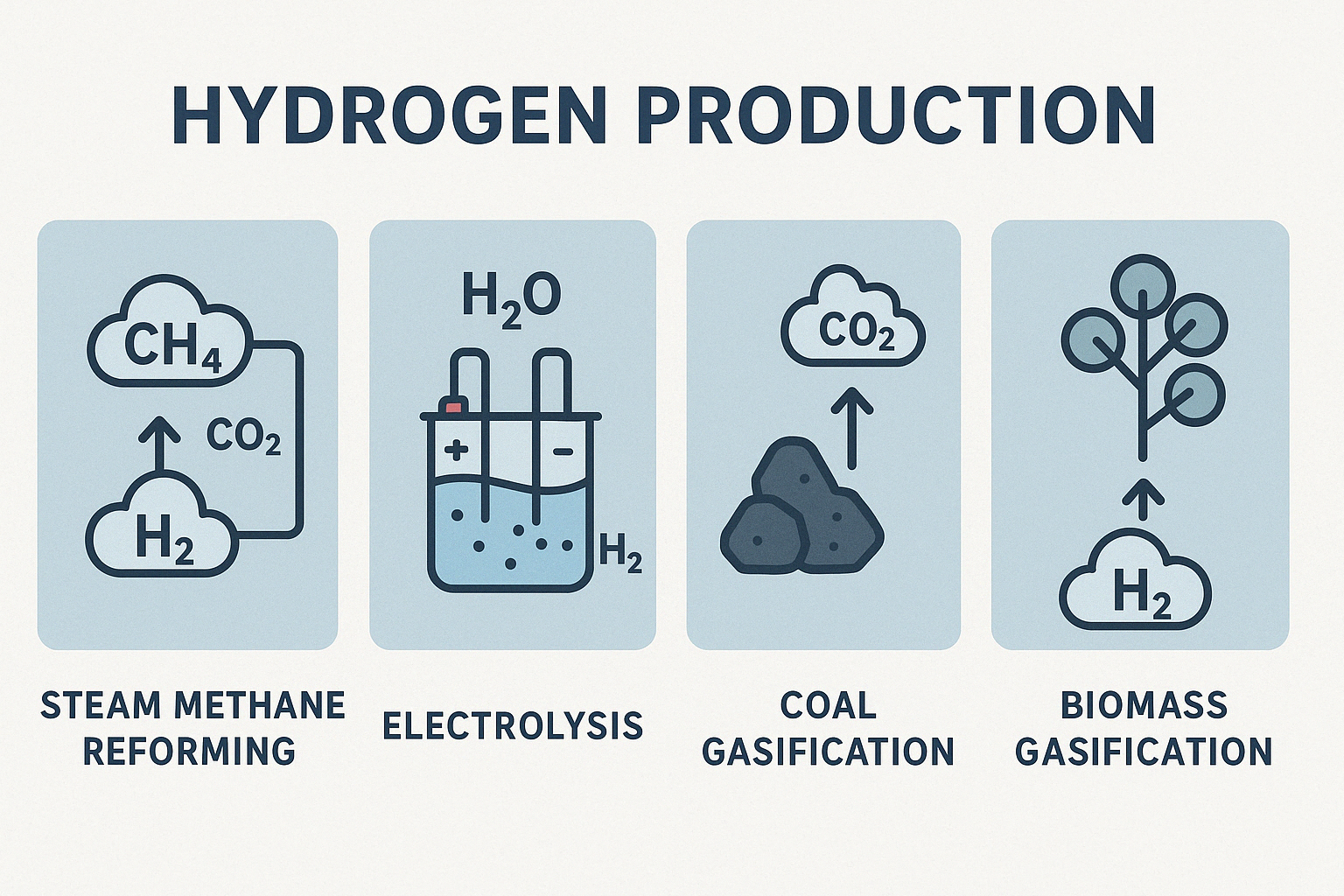
Hydrogen production technologies are methods used to produce hydrogen gas, a crucial energy carrier and component in many industrial processes. These technologies can be categorized into:
- Steam Methane Reforming (SMR): Extracts hydrogen from natural gas by reacting methane (CH₄) with steam at high temperatures. Most common but emits CO₂.
- Electrolysis: Splits water using electricity. If powered by renewables, it becomes green hydrogen. Our research explores catalysts like electrocatalysts, photocatalysts, and electrophoto catalysts to improve efficiency.
- Coal Gasification: Converts coal into hydrogen and other gases. Effective but emits CO₂.
- Biomass Gasification: Uses organic waste (wood, algae, etc.). More sustainable if responsibly sourced.
- Pyrolysis: An emerging method that decomposes methane into hydrogen and solid carbon without CO₂ emissions.
Among these, green hydrogen via electrolysis is the most sustainable future direction.
Carbon Capture, Utilization, and Storage (CCUS)
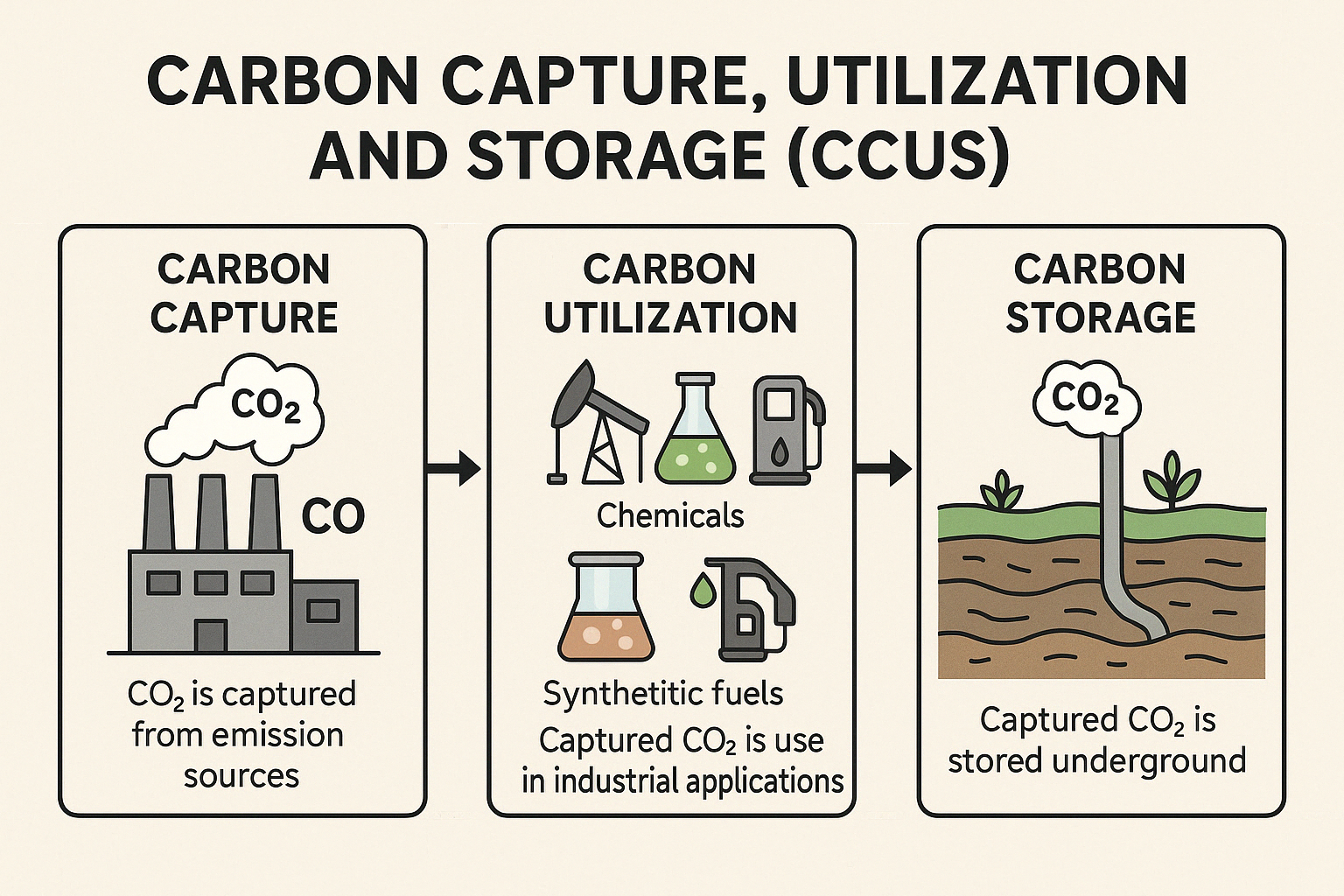
Carbon Capture, Utilization, and Storage (CCUS) refers to a set of technologies designed to reduce carbon dioxide (CO₂) emissions from industrial processes and power generation by capturing CO₂ before it is released into the atmosphere, utilizing it for other purposes, or storing it underground.
Carbon Capture: CO₂ is captured from emission sources like power plants, industrial facilities, or even directly from the air using various methods such as post-combustion capture, pre-combustion capture, and oxy-fuel combustion. The captured CO₂ is then compressed for transportation.
Carbon Utilization: The captured CO₂ can be used in several industrial applications, such as enhanced oil recovery, the production of chemicals, synthetic fuels, or materials like concrete and plastics. This process reduces the amount of CO₂ that would otherwise be released back into the atmosphere.
CO₂ Conversion Methods
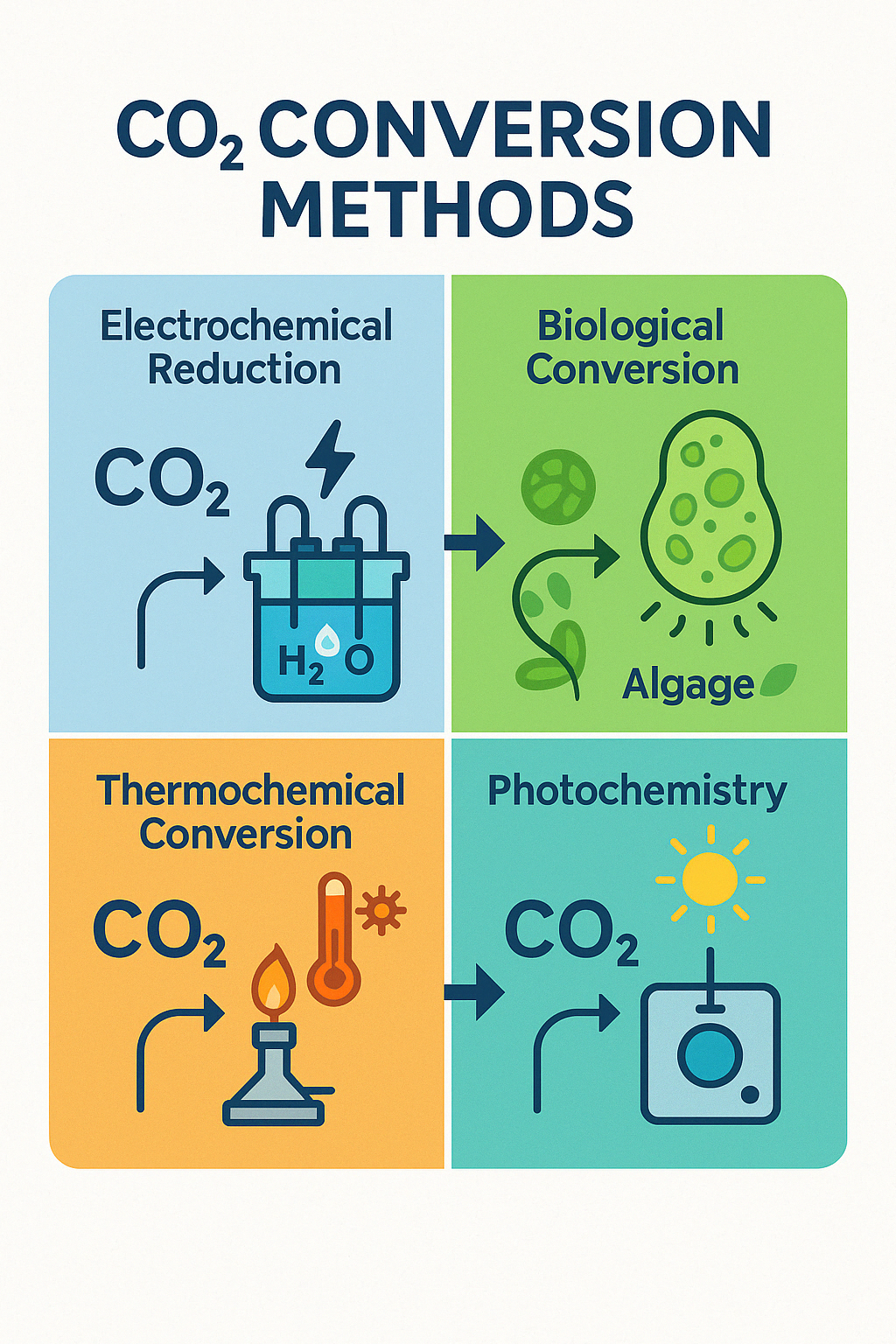
CO₂ Conversion Methods: These methods involve converting CO₂ into valuable products through chemical, biological, or physical processes. Key methods include:
- Electrochemical Reduction: Uses electricity to convert CO₂ into useful chemicals like methanol or formic acid.
- Biological Conversion: Employs microorganisms or algae to transform CO₂ into biofuels or biomass.
- Thermochemical Conversion: Applies heat and chemical reactions to produce synthetic fuels or chemicals.
- Photochemistry: Utilizes light, typically solar energy, to drive chemical reactions that reduce CO₂.
Fuel Cells
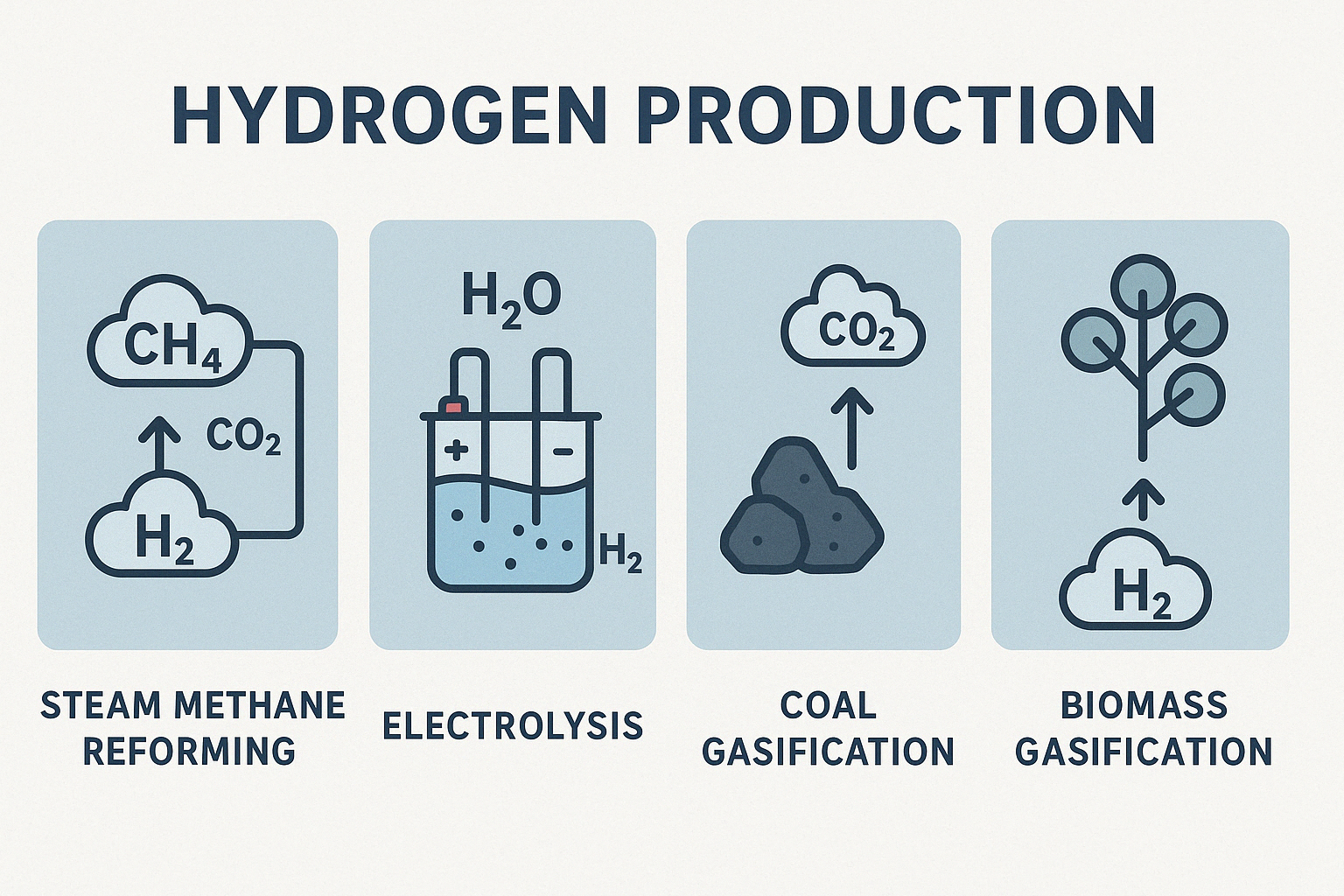
Fuel cells are electrochemical devices that convert chemical energy from a fuel (typically hydrogen) directly into electrical energy, through a reaction with oxygen or another oxidizing agent. This process is clean, as it produces electricity, heat, and water as byproducts, with little to no harmful emissions.
There are different types of fuel cells, based on the electrolyte used:
- Proton Exchange Membrane Fuel Cells (PEMFC): Used in transportation (like hydrogen-powered vehicles) due to quick start-up and high power density. They use a solid polymer membrane as the electrolyte.
- Solid Oxide Fuel Cells (SOFC): Used for stationary power generation. These operate at high temperatures with a ceramic electrolyte. More efficient but slower to start up.
- Alkaline Fuel Cells (AFC): Use an alkaline solution as the electrolyte. Known for high efficiency. Mainly used in space applications.
- Phosphoric Acid Fuel Cells (PAFC): Use liquid phosphoric acid. Applied in commercial and industrial settings.
- Molten Carbonate Fuel Cells (MCFC): High-temperature fuel cells used in large-scale, stationary power generation.
Fuel cells are considered a clean and efficient energy source—especially when hydrogen is used—making them a key technology in the transition to sustainable energy systems.
Advanced EV Battery Research
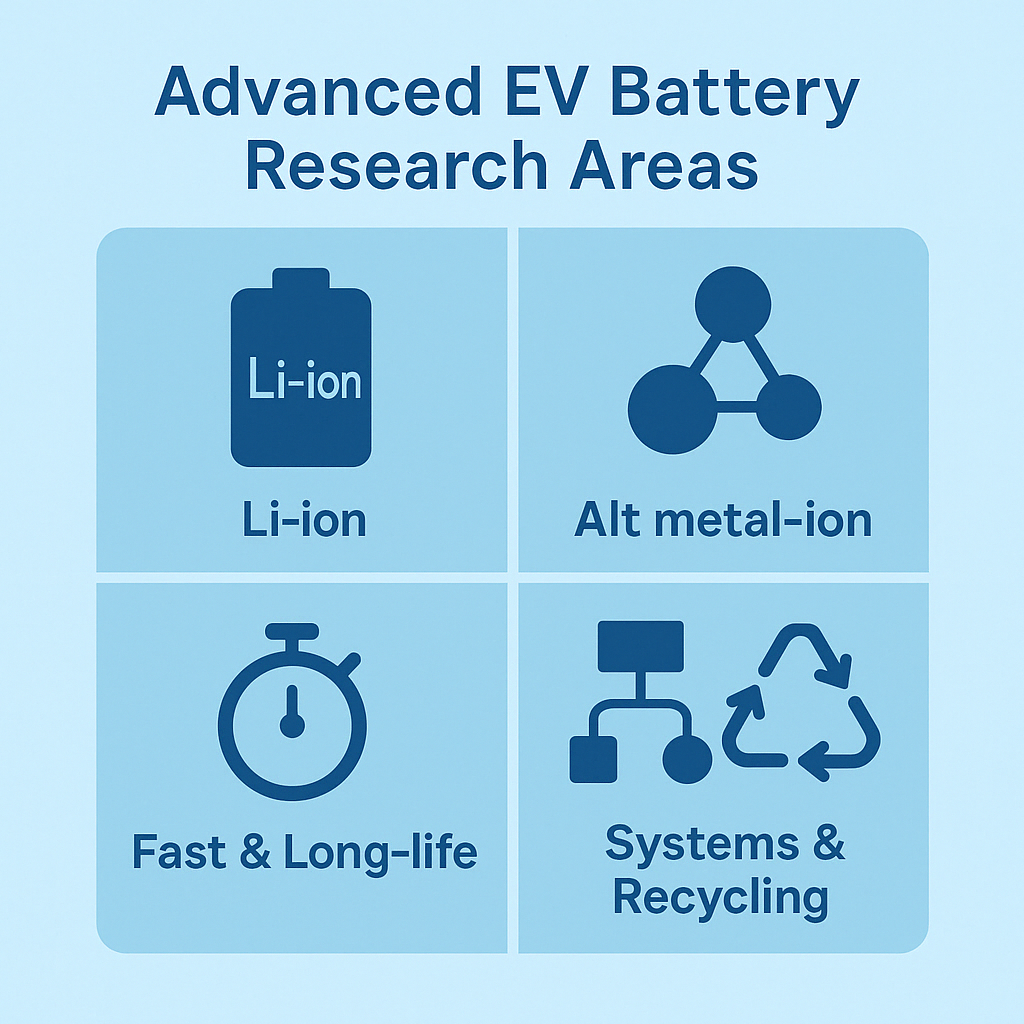
Our research on advanced EV batteries spans enhancements to conventional lithium-ion systems—such as high-nickel cathodes, silicon-rich anodes, and solid-state electrolytes—through exploration of alternative metal-ion chemistries (sodium-ion, magnesium- and aluminum-ion, lithium-sulfur, and zinc-ion), development of ultrafast and long-life architectures (lithium-titanate cells and hybrid capacitor–battery pairings), and holistic systems engineering that integrates next-generation battery-management and thermal-control strategies with sustainable sourcing and closed-loop recycling of critical materials.
Surface Engineering for Electrochemical Devices
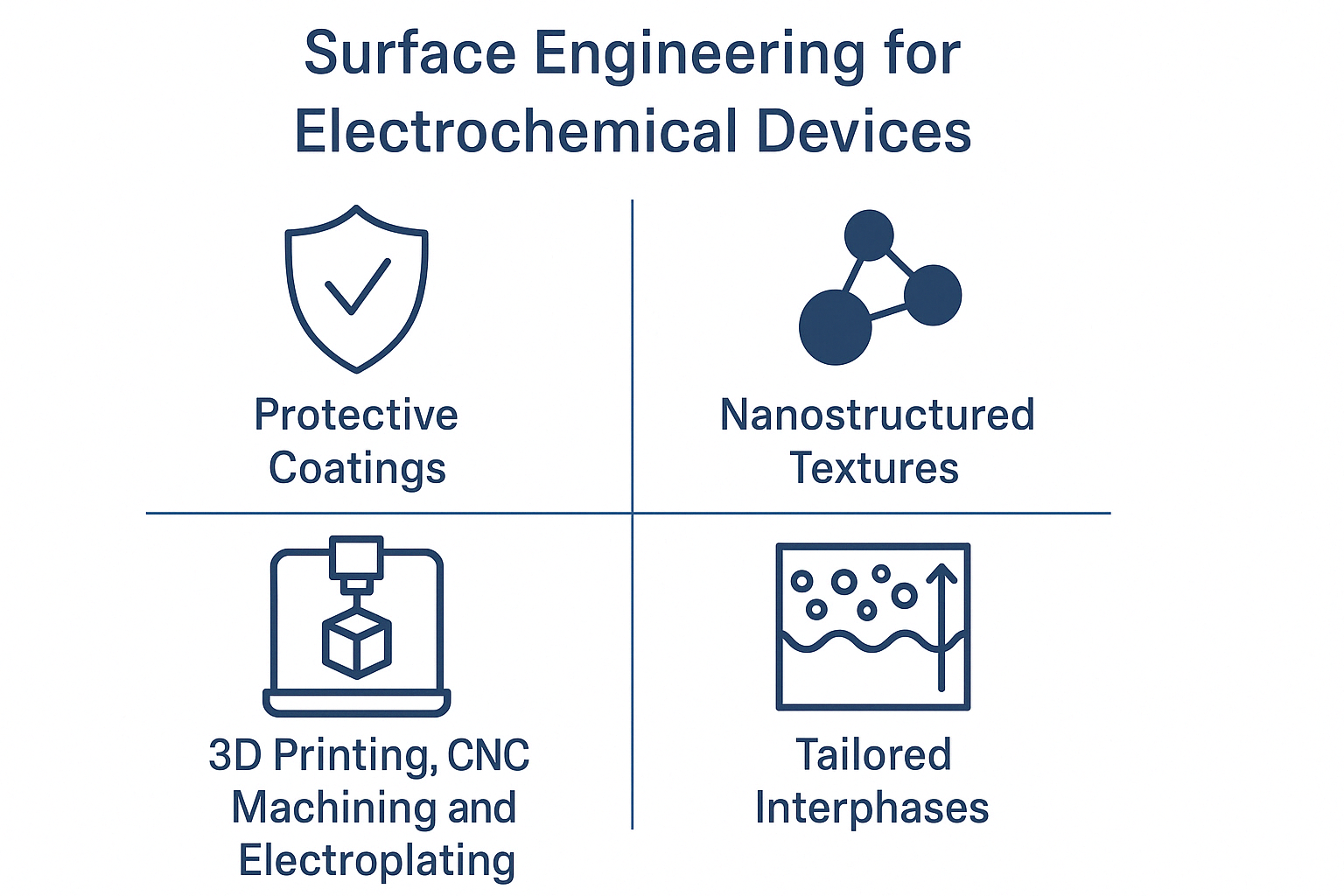
Our research explores advanced surface engineering techniques to enhance the performance and reliability of battery materials and electrochemical devices. By designing micro- and nanoscale surface features—such as protective coatings, nanostructured textures, and tailored interphases—we aim to improve ion/electron transport, increase stability, suppress dendrite formation, and optimize electrochemical kinetics.
We employ fabrication methods such as 3D printing, CNC machining, and electroplating to modify electrode surfaces, stabilize solid–electrolyte interphases (SEI), and boost catalytic activity in systems like Li-ion, Zn-ion, and solid-state batteries, as well as fuel cells and CO₂ reduction devices. This research supports the development of high-efficiency, long-life, and safe energy systems through interfacial and material innovation.